Radiation, Smoking, and Lung Cancer
A binational study provides new insights into the effects of smoking and radiation exposure on different histological types of lung cancer.
by Kiyohiko Mabuchi, RERF Department of Epidemiology, Charles E Land, RERF consultant, and health statistician, US National Cancer Institute, Radiation Epidemiology Branch, Bethesda, Maryland, and Suminori Akiba, RERF Department of Epidemiology.
This article was originally published in RERF Update 3(4):7-8, 1991.
Lung cancer is one of the leading forms of cancer in many countries. Cigarette smoking is considered to be the most important cause of lung cancer, whereas occupational exposures represent a less frequent but significant cause. Epidemiologic evidence also suggests that nutritional factors and certain host characteristics may modify an individual’s risk of developing lung cancer. Ionizing radiation has long been known to be capable of causing lung cancer, and it is an important cause in some populations, including the A-bomb survivors. In addition to the multiplicity of etiologic factors involved, lung cancer is characterized by different cell types in which it is manifested. These features complicate risk assessment because due consideration must be given to possible confounding and modifying effects of nonradiation factors. At the same time, they present an ideal prototype for studying multifactorial aspects of cancer induction involving radiation and more common cancer-causing agents, such as cigarette smoking.
How does smoking affect risk estimation?
The analysis of the most recent incidence data for 1950-1987 based on the tumor registries shows that lung cancer has one of the higher relative risks associated with radiation exposure. It is estimated that almost one-fifth of the observed exposed lung cancer cases are attributed to exposure to the A-bombings. The estimated excess relative risk (ERR) at 1 Sv is 0.95 and a significant dose-response relationship is demonstrated. A striking finding is that, among the usual risk modifiers such as age at exposure, attained age, sex, and time since exposure, sex has the strongest effect: Women have an estimated ERR of 1.93, about 4 times as high as that of men (0.48). This sex differential is largely explained by the difference in background cancer incidence between the sexes. The background male incidence is almost 3 times as high as the female incidence, most likely reflecting the higher prevalence of smoking in men than in women. Previously, K Kopecky et al (RERF TR 13-86) demonstrated that the sex difference in the relative risk of lung cancer in the A-bomb survivors diminished after adjustment had been made assuming additivity of the effect of smoking and radiation exposure (Figure).
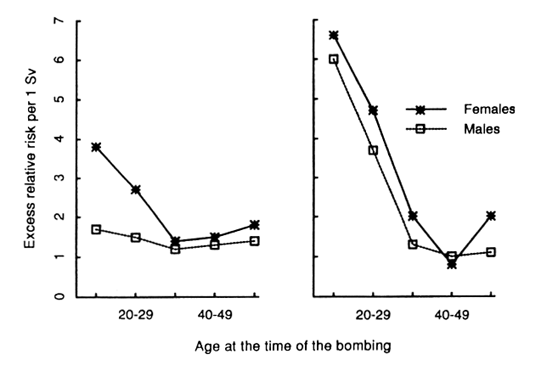
Figure. The excess relative risk of lung cancer per 1 Sv not adjusted for the additive effect of smoking (left) and adjusted assuming additive effect of smoking (right). (Redrawn from K Kopecky et al, RERF TR 13-86.)
How does smoking interact with radiation exposure?
It is clear that smoking and radiation have a combined effect on lung cancer. What is less clear is the precise manner in which these 2 factors interact with each other. Although an additive effect was suggested by Kopecky et al, the BEIR IV Committee noted that neither an additive nor a multiplicative model of interaction could be rejected on statistical grounds based on data from a case-control study of the A-bomb survivors (Health effects of exposure to low levels of ionizing radiation [BEIR V Report], Washington, DC, National Academy Press, 1990). Findings from studies of US uranium miners have been interpreted as supporting a multiplicative model for the interaction between cigarette smoking and exposure to radon progeny. According to the BEIR IV Committee’s analysis, a submultiplicative model may provide a more accurate description of the underlying relationship.
An accurate description of the statistical relationship is important not only for those concerned with risk assessment but also for those interested in mechanisms of radiation-induced cancer. For example, the presence of a multiplicative effect implies a synergism and suggests the presence of a biological interaction between the 2 factors. A better understanding of the association between smoking and lung cancer is also essential. For example, in Japan the relative risk of lung cancer associated with cigarette smoking has been much lower than that reported for the United States. This may be rather surprising in view of the widespread smoking habits prevailing in Japan. The explanation has been offered that the lack of cigarettes during World War II resulted in a 20- to 30-year lag in the nationwide adoption of smoking habits (EL Wynder et al, Cancer 67:746-63, 1991). However, the relative risk has begun to increase in more recent birth cohorts, ie, those who were still young when the war ended (S Akiba and T Hirayama, Environ Health Perspect 87:19-26, 1990). It will be interesting to observe the effect of this change on future radiation-smoking interaction analyses of the A-bomb survivor data.
What do different histological types mean?
Lung cancer can be categorized into 3 major cell types: squamous cell carcinoma, small cell carcinoma, and adenocarcinoma. It has been shown that cigarette smoking is related to all 3 types of cancer, although the relationship is stronger for the squamous cell and small cell types than adenocarcinoma. In the A-bomb survivors, radiation exposure was found to increase the risk for all 3 major types of lung cancer also, but the risk for small cell carcinoma appeared to be greater than that for the other 2 types. Preliminary results from a recently completed binational study of lung cancer in US uranium miners and Japanese A-bomb survivors provide new insights into the interrelationship among smoking, radiation dose, and radiation quality as they relate to histologic presentations of cancer. The objectives of this study were, first, to determine whether there are differences in histologic types of lung cancer between the 2 populations, and, second, to assess how differences in radiation exposure and other factors can explain any observed difference in histologic presentation of lung cancer. Previous studies by Gino Saccomanno and his colleagues (V Archer et al, Cancer 34:2056, 1974; G Saccomanno et al, Cancer62:1402-8, 1988) had suggested that the frequency of small cell carcinoma might be unusually high among the heavily exposed uranium miners.
Comparison of the 2 populations is of special interest because they have distinctly different radiation-exposure characteristics: The uranium miners were exposed to nonpenetrating alpha radiation from inhaled radon progeny over a prolonged period of time, whereas the A-bomb survivors were almost instantaneously exposed to mostly penetrating gamma rays from an external source. The radon progeny attach to dust particles which tend to be deposited in the large and medium bronchi, whereas the smaller bronchi–having a larger aggregate surface area–received most of the bronchial radiation from A-bomb exposure. Since squamous cell and small cell carcinomas originate mainly in the large and medium bronchi (adenocarcinoma is more likely to occur in the small bronchi), differences in histologic types between the 2 populations might be expected on radiobiological grounds.
To conduct a comparison in a controlled and standardized manner, a binational panel of pathologists, 4 Americans headed by Gino Saccomano (St Mary’s Hospital, Grand Junction, Colo) and 4 Japanese led by Yukio Shimosato (Japanese National Cancer Center, Tokyo), reviewed pathology specimens available for 108 lung cancer patients from the Life Span Study cohort of A-bomb survivors and 92 uranium miners with lung cancer studied in Colorado. To the extent practicable, the cases were selected from each population to be evenly divided between high and low doses. Histologic diagnoses were classified using the WHO classification for pulmonary neoplasms. As expected from the earlier studies, the relative frequencies of lung cancer classified into the 3 main types were indeed different in the 2 populations. Most of the uranium miner cases, but only one-eighth of the A-bomb survivor cases, had small cell carcinoma. Nearly half of the A-bomb cases, but only less than one-tenth of the miner cases, had adenocarcinoma; squamous cell carcinoma made up about one-third of both series.
The exposures of the cases selected from the 2 populations were such that far more of the uranium miner cases than A-bomb survivor cases were likely to have been caused by radiation exposure, based on published dose-response estimates (Health risks of radon and other internally deposited alpha particle emitters [BEIR IV Report], Washington, DC, National Academy Press, 1988; BEIR V Report, 1990; Y Shimizu et al, Radiation Research 121:120-41, 1990). The only female cases were among the A-bomb survivors, and there were also differences with respect to age and year of diagnosis. Male A-bomb survivors smoked about as much, on the average, as uranium miners, but the proportion of female smokers, and the amount smoked per smoker, was substantially less among the female survivors. The analytical problem for the investigators was to determine how much of the observed population difference with respect to histological type could be explained in terms of the variables just mentioned and how much could not be explained and therefore might plausibly be attributed to population differences, including radiation quality and internal vs external sources of exposure.
As expected, squamous cell carcinomas occurred disproportionately among smokers, and their relative frequency increased by cumulative number of cigarette pack years, with or without adjustment for age, calendar year, sex, population, or radiation exposure. The relative frequencies of small cell carcinoma and adenocarcinoma were markedly different in the 2 populations, as discussed above, and remained so even after adjustment for age and year of diagnosis, sex, and smoking history. Somewhat surprisingly, however, the difference was drastically reduced by adjustment for radiation dose, leaving essentially no apparent population difference to be explained by factors not included in the analysis. Thus it appeared that the difference in histological type distribution between populations can be attributed to the fact that far more of the miner cases were radiation-related, and it was not necessary to suppose that different types of radiation or different modes of exposure result in different types of lung cancer.
The adjustment for “radiation dose” mentioned in the previous paragraph was actually in terms of estimated radiation-related risk, ie, the estimated probability that a given A-bomb survivor or uranium miner case was caused by the exposure received. When the individual exposure experiences in the 2 populations were evaluated on this common scale, the distribution of histological types appeared to conform to a single pattern in which, regardless of population, radiation-induced cancers tended disproportionately to be of the small cell type, and nonradiation-related lung cancers were correspondingly more likely to be adenocarcinoma.
Many questions still remain to be resolved in this interesting area of research. Will the nature of interactive effect between cigarette smoking and radiation exposure change in the A-bomb survivors as younger birth cohorts with more intense smoking exposure enter ages of high cancer rate? How does the cessation of smoking modify the interactive effect? Does the sequence of exposure events make any difference? These are some of the important epidemiologic questions. However, understanding the underlying biological mechanisms will require more than just answering these questions. For example, RERF is currently involved in another collaborative study, also focusing on lung cancer and also contrasting the A-bomb survivors with another population, that seeks to identify, in certain tumor suppressor genes, “molecular fingerprints” that may be unique to ionizing radiation or to other carcinogens such as alkylating agents.